The end goal of our research is leveraging microbial communities to protect public health and the environment, generate renewable energy, and recover valuable resources from wastewater and other low-value societal “wastes”. We ask how complex microbial communities assemble, fluctuate over time and space, and perform useful functions, then put these microbial communities to work. Our research is inherently interdisciplinary and requires integration of molecular biology and bioinformatics, microscopy tools, environmental bioprocess development and modeling, and classical microbiology techniques. Much of our work targets novel applications of complex microbial consortia for wastewater and water treatment. Specific research projects in the Wells group include:
Innovative and sustainable biological wastewater treatment processes
Modern wastewater management is vital for protecting human and environmental health. Traditional wastewater treatment uses an unsustainable “once-through” model that focuses on waste removal and disposal. This strategy has led to many negative side effects including the emission of nitrogen and phosphorus to the environment, greenhouse gas emissions, and high treatment costs that will intensify due to urbanization and population growth. Our group integrates microbial ecology with wastewater engineering to develop new mixed culture processes for complete nutrient removal. We are pursuing novel nutrient removal strategies such as Coupled Aerobic-anoxic Nitrous Decomposition Operation (CANDO). CANDO combines nitrous oxide generation for energy recovery and complete nutrient removal in a single bioprocess. By combining nitrogen and phosphorus removal, CANDO lowers the land usage and operating costs for nutrient removal and can recover phosphorus, a nonrenewable resource.
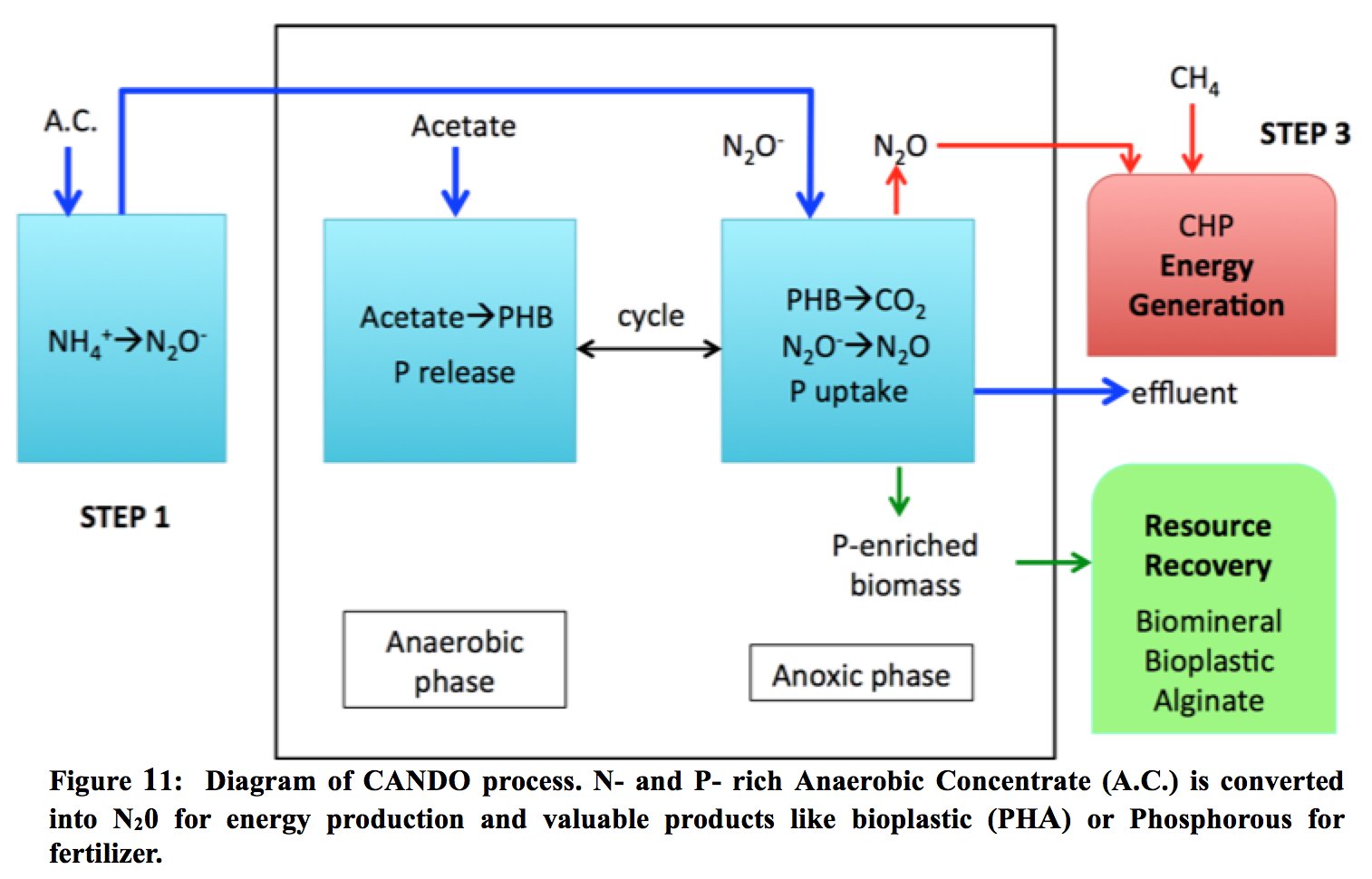
By recovering nitrous oxide (N2O), a powerful oxidant that can supercharge existing biogas streams, CANDO brings wastewater treatment closer to energy independence. We are currently trying to maximize N2O production and N and P removal with denitrifying polyphosphate accumulating organisms (DPAOs) in a lab-scale reactor. Reactor performance data is being used to develop a metabolic model that describes the key biochemical reactions for DPAO N2O production. Modeling efforts could help define the parameter space for maximum regions of N2O production as well as find ways to reduce unwanted N2O production in traditional denitrification processes. It may also help elucidate complex biochemical reactions, microbial activities and mechanisms for N2O production.
Microbial ecology of engineered and impacted natural environments
Our group is interested in understanding the forces that shape microbial communities in engineered, freshwater and soil communities to improve aquatic ecosystem health and wastewater treatment performance. The development of high-throughput sequencing has given microbiologists unprecedented ability to ask, “Who is present in microbial communities and what are they doing?” Amplicon and metagenomic sequencing have been used to investigate communities from environments as diverse as the human gut to Antarctic permafrost.
Analyzing the ever-growing datasets produced by sequencing technologies continues to be a challenge. Working with the Metropolitan Water Reclamation District, we are developing computational tools to predict seasonal community changes and identify environmental conditions that lead to settling and de-ammonification upsets in full-scale plants. We’re also looking for sources of antibiotic resistance and production genes in Midwest waterways with professors Brian Murphy and Rachel Poretsky at UIC.

Microbial nitrogen cycling in natural and engineered environments
Nitrogen discharges due to human activities greatly increase primary biological productivity in marine environments and have emerged as the single greatest pollution problem in the coastal waters of the United States. This phenomenon, referred to as eutrophication, leads to hypoxic conditions (dissolved oxygen concentrations less than 3 mg/L), habitat destruction, and a loss of biodiversity in affected water bodies. Municipal wastewater is a significant source of bioavailable nitrogen, and wastewater treatment plants (WWTPs) are under increasing pressure to implement nitrogen removal processes while minimizing costs and greenhouse gas emissions.Nitrification/denitrification has been the principal technology to remove nitrogen from municipal wastewater. Using this technology, influent nitrogen consisting primarily of ammonium (NH4+) is aerobically oxidized first to nitrite (NO2-), followed by oxidation of the nitrite to nitrate (NO3-) (nitrification). Nitrification is followed by the anoxic reduction of nitrate to molecular nitrogen (N2) using biodegradable carbon as the reductant (denitrification). However, the energy and material inputs required to support nitrification/denitrification dramatically increase the operating costs and environmental impact of wastewater treatment.An alternative process, partial nitritation followed by anaerobic ammonium oxidation (anammox), has emerged as a more resource efficient pathway to convert ammonium to molecular nitrogen in WWTPs. Nitrogen removal via anammox processes requires no organic carbon, and significantly reduces carbon dioxide emissions and aeration energy requirements compared to nitrification/denitrification. The impact of this technology on water pollution control has been enormous. Anammox, operated in conjunction with partial nitritation, is a state of the art technology for nitrogen removal from warm, high ammonium concentration waste streams such as anaerobic digester effluent, agro-industrial waste streams, and landfill leachate.
The leading edge of anammox research is the application of partial nitritation/anammox to the colder, low ammonium strength wastewater encountered in the mainstream of municipal WWTPs, and in collaboration with the Metropolitan Water Reclamation District of Greater Chicago (MWRDGC), our group is working to reach this goal.
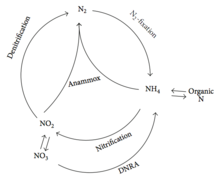

The leading edge of anammox research is the application of partial nitritation/anammox to the colder, low ammonium strength wastewater encountered in the mainstream of municipal WWTPs, and in collaboration with the Metropolitan Water Reclamation District of Greater Chicago (MWRDGC), our group is working to reach this goal.
Bioenergy production and resource recovery from wastewater and other low value feed streams
Recovering energy, fuel or platform chemicals by integrating waste streams in a systems view of nutrient management is a critical goal identified by the EPA. Microbial fuel cells and electrolysis cells (MFCs and MECs) have been demonstrated as potential routes to energy, hydrogen, or hydrogen peroxide (H2O2) production from municipal wastewater. MFCs rely on extracellular electron transfer to simultaneously treat wastewater while generating electrical current. Hydrogen peroxide production is especially attractive because it can be used onsite as a dilute solution for disinfection or other industrial processes and it is traditionally produced via the energy-intensive anthraquinone processs. MEC-derived H2O2 may also be a cost-effective oxidant for depolymerizing lignin, a major component of lignocellulosic waste biomass. We are developing and characterizing an integrated and sustainable system for simultaneous production of high value chemicals (H2O2 and aromatic monomers) and clean water from wastewater and waste lignin.

Biofilm structure function relationships and biofilm reactors
Biofilms are complex communities of aggregated cells that attach to surfaces and develop complex morphological characteristics, stratified microenvironments, and cell-to-cell signaling capabilities. The structure and composition of biofilms in natural environments result from a wide range of interconnected ecological factors such as flow conditions, nutrient availability, and microbial diversity. Biofilm scan have beneficial uses, particularly for pollution control in natural and engineered aquatic environments. Biofilm formation can also have negative consequences in certain environments, such as plaque on teeth, cellular buildup on implanted medical devices, and fouling of pipes. Currently in the Wells lab, a variety of biofilms are being researched including heterotrophic mixed-species biofilms in rotating annular reactors and moving bed biofilm reactors as well as single and dual species biofilms in flow cells.
